- Axial
- Posts
- Electrically driven proton transfer promotes Brønsted acid catalysis by orders of magnitude
Electrically driven proton transfer promotes Brønsted acid catalysis by orders of magnitude
Inventors & their inventions
Axial: https://linktr.ee/axialxyz
Axial partners with great founders and inventors. We invest in early-stage life sciences companies such as Appia Bio, Seranova Bio, Delix Therapeutics, Simcha Therapeutics, among others often when they are no more than an idea. We are fanatical about helping the rare inventor who is compelled to build their own enduring business. If you or someone you know has a great idea or company in life sciences, Axial would be excited to get to know you and possibly invest in your vision and company. We are excited to be in business with you — email us at [email protected]
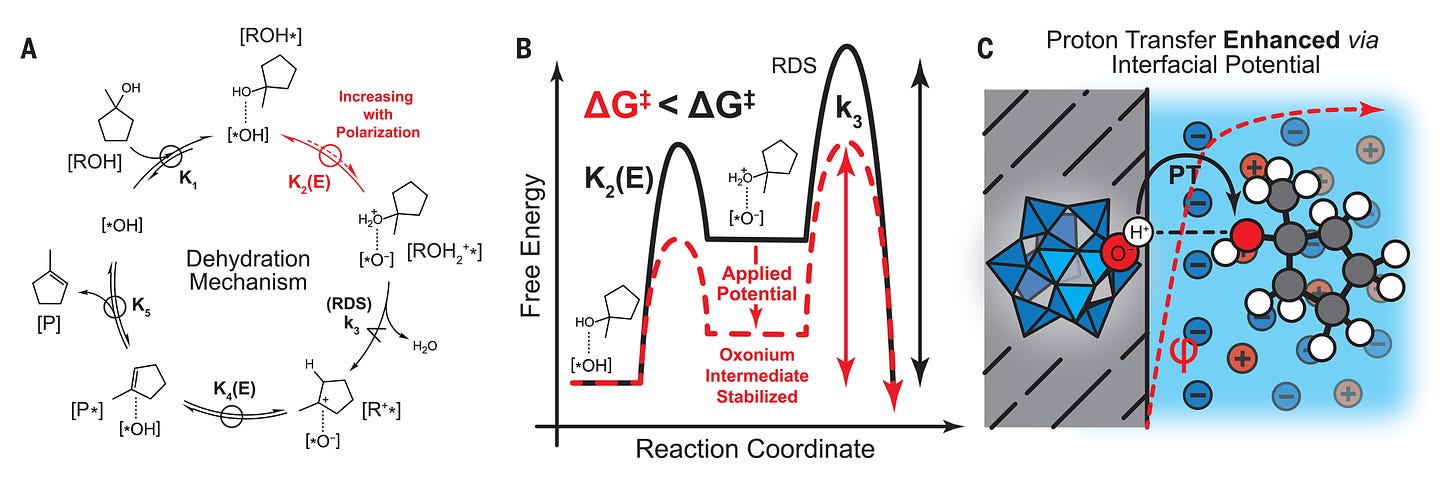
The research explores the fascinating relationship between electric fields and Brønsted acid catalysis, a crucial reaction type in chemistry and biology. The study demonstrates that varying the surface electrochemical potential of Brønsted acid catalysts can dramatically enhance reaction rates, potentially revolutionizing the field of heterogeneous catalysis.
The authors begin by outlining the known roles of electric fields in both biological and electrochemical systems. In biological systems, enzymes utilize precisely placed charged amino acid residues to create strong, oriented electric fields that promote catalysis. Electrochemical systems, on the other hand, generate an electric field at the metal/solution interface due to the accumulation of opposing ionic charge in solution. This electric field significantly impacts the rates of electrochemical half-reactions by influencing the free energy change associated with electron transfer to or from the electrode.
The research presented in this paper explores a unique aspect of catalysis where the influence of electric fields on reaction rates is not fully understood: the case of redox-neutral thermochemical reactions. These reactions, unlike their electrochemical counterparts, do not involve the net exchange of electrons. As such, it is not clear how manipulating surface potential would affect their rate. To address this gap, the authors focus on Brønsted acid catalyzed reactions, proposing that variations in applied potential could act to augment the rate of these reactions by modulating proton transfer steps that are intrinsically sensitive to electric fields.
The study focuses on the dehydration of 1-methylcyclopentanol catalyzed by carbon-supported phosphotungstic acid (PTA) as a model system. Through a combination of experimental and theoretical approaches, the authors demonstrate that varying the applied potential of this catalyst results in a remarkable 100,000-fold rate enhancement for this reaction. This significant rate increase occurs due to a change in the catalyst’s effective acidity, which is controlled by the interfacial electrostatic potential drop across the catalytic interface. The authors further confirm that this phenomenon is not restricted to the specific dehydration reaction studied, finding similar trends for both the dehydration reaction catalyzed by Ti/TiOyHx and for a Friedel-Crafts acylation reaction. These findings highlight the universal nature of the potential-dependent Brønsted acid catalysis effect.
A key contribution of this work is the development of a mechanistic model that explains the observed rate enhancements. The authors propose that the strong dependence of the reaction rate on potential arises from an electrically driven shift in the pre-equilibrium proton transfer between the catalyst and the reactant molecule. They suggest that positive polarization promotes the transfer of a proton from the catalyst to the substrate molecule, ultimately accelerating the rate-limiting C-O bond cleavage step and driving the overall reaction.
The authors then present further evidence to validate this mechanistic model by investigating the impact of electrolyte concentration on the reaction rate. They show that the observed rate enhancement is not simply a function of the absolute value of the potential at the surface but is more closely related to the gradient of the potential within the electric double layer. This observation reinforces the idea that the proton transfer step is influenced by the electric field strength, and that the kinetic enhancement is not solely due to a change in the overall free energy of the reaction.
To further expand their study, the authors examine the catalytic activity of a different Brønsted acid catalyst, namely Ti/TiOyHx. Their results confirm that the potential-dependent enhancement of Brønsted acid catalysis is not specific to the PTA/C system. The authors find that increasing the potential of a Ti foil electrode by just 120 mV leads to a 16-fold increase in the rate of the 1-methylcyclopentanol dehydration reaction. This outcome supports the broader applicability of the proposed mechanism and underscores the universality of the potential-driven promotion effect.
Finally, the authors explore the potential-dependent behavior of a different type of Brønsted acid catalyzed reaction: the Friedel-Crafts acylation of anisole with acetic anhydride. They observe that the rate of this reaction increases by four orders of magnitude over a potential range of just 45 mV, demonstrating the potential of this approach to significantly enhance various other Brønsted acid catalyzed reactions.
The authors conclude by highlighting the importance of the interfacial potential in driving Brønsted acid catalysis. They emphasize that this previously underappreciated parameter can be exploited to enhance the activities of Brønsted acid catalysts, potentially leading to significant advancements in heterogeneous catalysis. The study suggests that careful control of the interfacial potential could offer a powerful means to achieve high reaction rates and develop more efficient and sustainable catalytic processes.
This research has several implications for the future of Brønsted acid catalysis. It provides a theoretical framework for understanding the influence of electric fields on reaction rates, paving the way for the development of novel strategies for enhancing catalytic activity. It also highlights the importance of carefully controlling the interfacial potential in designing and optimizing Brønsted acid catalyzed reactions, which could lead to new and improved catalysts for a wide range of applications. The authors acknowledge the need for further research to fully elucidate the complex mechanisms underlying the potential-dependent behavior of Brønsted acid catalysts, but the findings of this study provide a compelling starting point for future investigations in this important area of catalysis.
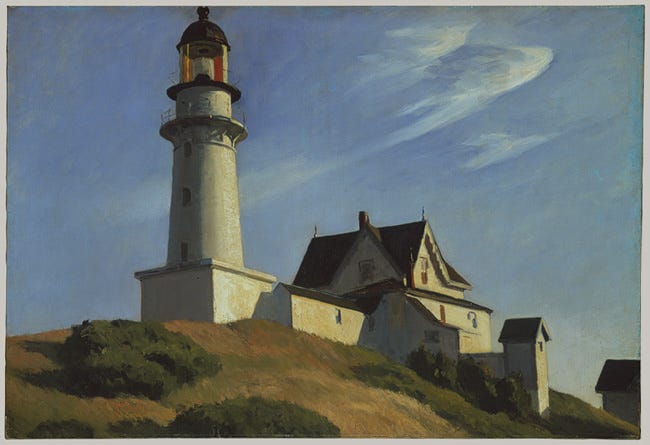